Inside the quest to map the universe with mysterious bursts of radio energy
When our universe was less than half as old as it is today, a burst of energy that could cook a sun’s worth of popcorn shot out from somewhere amid a compact group of galaxies. Some 8 billion years later, radio waves from that burst reached Earth and were captured by a sophisticated low-frequency radio telescope in the Australian outback.
The signal, which arrived on June 10, 2022, and lasted for under half a millisecond, is one of a growing class of mysterious radio signals called fast radio bursts. In the last 10 years, astronomers have picked up nearly 5,000 of them. This one was particularly special: nearly double the age of anything previously observed, and three and a half times more energetic.
But like the others that came before, it was otherwise a mystery. No one knows what causes fast radio bursts. They flash in a seemingly random and unpredictable pattern from all over the sky. Some appear from within our galaxy, others from previously unexamined depths of the universe. Some repeat in cyclical patterns for days at a time and then vanish; others have been consistently repeating every few days since we first identified them. Most never repeat at all.
Despite the mystery, these radio waves are starting to prove extraordinarily useful. By the time our telescopes detect them, they have passed through clouds of hot, rippling plasma, through gas so diffuse that particles barely touch each other, and through our own Milky Way. And every time they hit the free electrons floating in all that stuff, the waves shift a little bit. The ones that reach our telescopes carry with them a smeary fingerprint of all the ordinary matter they’ve encountered between wherever they came from and where we are now.
This makes fast radio bursts, or FRBs, invaluable tools for scientific discovery—especially for astronomers interested in the very diffuse gas and dust floating between galaxies, which we know very little about.
“We don’t know what they are, and we don’t know what causes them. But it doesn’t matter. This is the tool we would have constructed and developed if we had the chance to be playing God and create the universe,” says Stuart Ryder, an astronomer at Macquarie University in Sydney and the lead author of the Science paper that reported the record-breaking burst.
Many astronomers now feel confident that finding more such distant FRBs will enable them to create the most detailed three-dimensional cosmological map ever made—what Ryder likens to a CT scan of the universe. Even just five years ago making such a map might have seemed an intractable technical challenge: spotting an FFB and then recording enough data to determine where it came from is extraordinarily difficult because most of that work must happen in the few milliseconds before the burst passes.
But that challenge is about to be obliterated. By the end of this decade, a new generation of radio telescopes and related technologies coming online in Australia, Canada, Chile, California, and elsewhere should transform the effort to find FRBs—and help unpack what they can tell us. What was once a series of serendipitous discoveries will become something that’s almost routine. Not only will astronomers be able to build out that new map of the universe, but they’ll have the chance to vastly improve our understanding of how galaxies are born and how they change over time.
Where’s the matter?
In 1998, astronomers counted up the weight of all of the identified matter in the universe and got a puzzling result.
We know that about 5% of the total weight of the universe is made up of baryons like protons and neutrons— the particles that make up atoms, or all the “stuff” in the universe. (The other 95% includes dark energy and dark matter.) But the astronomers managed to locate only about 2.5%, not 5%, of the universe’s total. “They counted the stars, black holes, white dwarfs, exotic objects, the atomic gas, the molecular gas in galaxies, the hot plasma, etc. They added it all up and wound up at least a factor of two short of what it should have been,” says Xavier Prochaska, an astrophysicist at the University of California, Santa Cruz, and an expert in analyzing the light in the early universe. “It’s embarrassing. We’re not actively observing half of the matter in the universe.”
All those missing baryons were a serious problem for simulations of how galaxies form, how our universe is structured, and what happens as it continues to expand.
Astronomers began to speculate that the missing matter exists in extremely diffuse clouds of what’s known as the warm–hot intergalactic medium, or WHIM. Theoretically, the WHIM would contain all that unobserved material. After the 1998 paper was published, Prochaska committed himself to finding it.
But nearly 10 years of his life and about $50 million in taxpayer money later, the hunt was going very poorly.
That search had focused largely on picking apart the light from distant galactic nuclei and studying x-ray emissions from tendrils of gas connecting galaxies. The breakthrough came in 2007, when Prochaska was sitting on a couch in a meeting room at the University of California, Santa Cruz, reviewing new research papers with his colleagues. There, amid the stacks of research, sat the paper reporting the discovery of the first FRB.
Duncan Lorimer and David Narkevic, astronomers at West Virginia University, had discovered a recording of an energetic radio wave unlike anything previously observed. The wave lasted for less than five milliseconds, and its spectral lines were very smeared and distorted, unusual characteristics for a radio pulse that was also brighter and more energetic than other known transient phenomena. The researchers concluded that the wave could not have come from within our galaxy, meaning that it had traveled some unknown distance through the universe.
Here was a signal that had traversed long distances of space, been shaped and affected by electrons along the way, and had enough energy to be clearly detectable despite all the stuff it had passed through. There are no other signals we can currently detect that commonly occur throughout the universe and have this exact set of traits.
“I saw that and I said, ‘Holy cow—that’s how we can solve the missing-baryons problem,’” Prochaska says. Astronomers had used a similar technique with the light from pulsars— spinning neutron stars that beam radiation from their poles—to count electrons in the Milky Way. But pulsars are too dim to illuminate more of the universe. FRBs were thousands of times brighter, offering a way to use that technique to study space well beyond our galaxy.

There’s a catch, though: in order for an FRB to be an indicator of what lies in the seemingly empty space between galaxies, researchers have to know where it comes from. If you don’t know how far the FRB has traveled, you can’t make any definitive estimate of what space looks like between its origin point and Earth.
Astronomers couldn’t even point to the direction that the first 2007 FRB came from, let alone calculate the distance it had traveled. It was detected by an enormous single-dish radio telescope at the Parkes Observatory (now called the Murriyang) in New South Wales, which is great at picking up incoming radio waves but can pinpoint FRBs only to an area of the sky as large as Earth’s full moon. For the next decade, telescopes continued to identify FRBs without providing a precise origin, making them a fascinating mystery but not practically useful.
Then, in 2015, one particular radio wave flashed—and then flashed again. Over the course of two months of observation from the Arecibo telescope in Puerto Rico, the radio waves came again and again, flashing 10 times. This was the first repeating burst of FRBs ever observed (a mystery in its own right), and now researchers had a chance to determine where the radio waves had begun, using the opportunity to home in on its location.
In 2017, that’s what happened. The researchers obtained an accurate position for the fast radio burst using the NRAO Very Large Array telescope in central New Mexico. Armed with that position, the researchers then used the Gemini optical telescope in Hawaii to take a picture of the location, revealing the galaxy where the FRB had begun and how far it had traveled. “That’s when it became clear that at least some of these we’d get the distance for. That’s when I got really involved and started writing telescope proposals,” Prochaska says.
That same year, astronomers from across the globe gathered in Aspen, Colorado, to discuss the potential for studying FRBs. Researchers debated what caused them. Neutron stars? Magnetars, neutron stars with such powerful magnetic fields that they emit x-rays and gamma rays? Merging galaxies? Aliens? Did repeating FRBs and one-offs have different origins, or could there be some other explanation for why some bursts repeat and most do not? Did it even matter, since all the bursts could be used as probes regardless of what caused them? At that Aspen meeting, Prochaska met with a team of radio astronomers based in Australia, including Keith Bannister, a telescope expert involved in the early work to build a precursor facility for the Square Kilometer Array, an international collaboration to build the largest radio telescope arrays in the world.
The construction of that precursor telescope, called ASKAP, was still underway during that meeting. But Bannister, a telescope expert at the Australian government’s scientific research agency, CSIRO, believed that it could be requisitioned and adapted to simultaneously locate and observe FRBs.
Bannister and the other radio experts affiliated with ASKAP understood how to manipulate radio telescopes for the unique demands of FRB hunting; Prochaska was an expert in everything “not radio.” They agreed to work together to identify and locate one-off FRBs (because there are many more of these than there are repeating ones) and then use the data to address the problem of the missing baryons.
And over the course of the next five years, that’s exactly what they did—with astonishing success.
Building a pipeline
To pinpoint a burst in the sky, you need a telescope with two things that have traditionally been at odds in radio astronomy: a very large field of view and high resolution. The large field of view gives you the greatest possible chance to detect a fleeting, unpredictable burst. High resolution lets you determine where that burst actually sits in your field of view.
ASKAP was the perfect candidate for the job. Located in the westernmost part of the Australian outback, where cattle and sheep graze on public land and people are few and far between, the telescope consists of 36 dishes, each with a large field of view. These dishes are separated by large distances, allowing observations to be combined through a technique called interferometry so that a small patch of the sky can be viewed with high precision.
The dishes weren’t formally in use yet, but Bannister had an idea. He took them and jerry-rigged a “fly’s eye” telescope, pointing the dishes at different parts of the sky to maximize its ability to spot something that might flash anywhere.
“Suddenly, it felt like we were living in paradise,” Bannister says. “There had only ever been three or four FRB detections at this point, and people weren’t entirely sure if [FRBs] were real or not, and we were finding them every two weeks.”
When ASKAP’s interferometer went online in September 2018, the real work began. Bannister designed a piece of software that he likens to live-action replay of the FRB event. “This thing comes by and smacks into your telescope and disappears, and you’ve got a millisecond to get its phone number,” he says. To do so, the software detects the presence of an FRB within a hundredth of a second and then reaches upstream to create a recording of the telescope’s data before the system overwrites it. Data from all the dishes can be processed and combined to reconstruct a view of the sky and find a precise point of origin.
The team can then send the coordinates on to optical telescopes, which can take detailed pictures of the spot to confirm the presence of a galaxy—the likely origin point of the FRB.
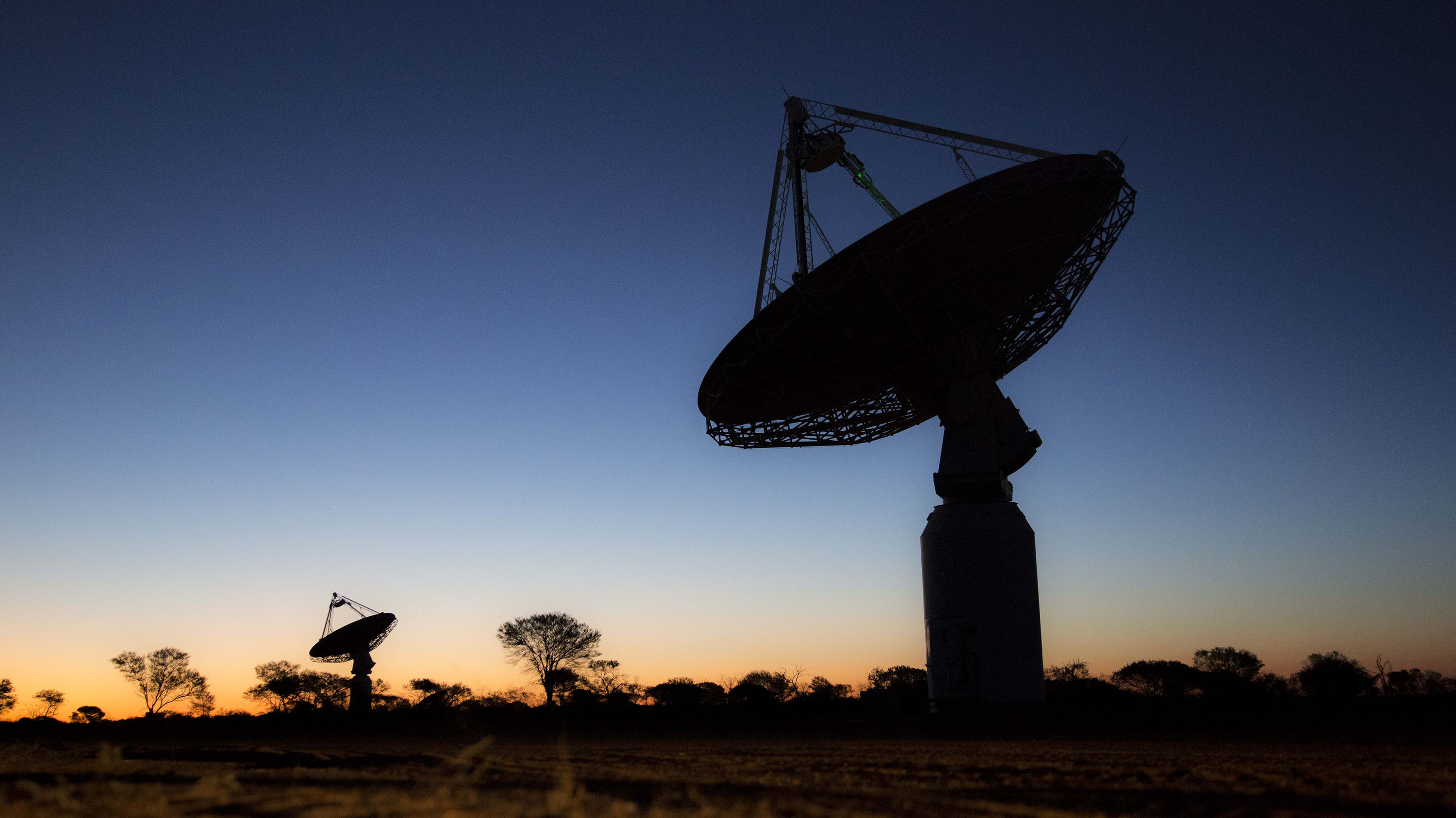
Ryder’s team used data on the galaxy’s spectrum, gathered from the European Southern Observatory, to measure how much its light stretched as it traversed space to reach our telescopes. This “redshift” becomes a proxy for distance, allowing astronomers to estimate just how much space the FRB’s light has passed through.
In 2018, the live-action replay worked for the first time, making Bannister, Ryder, Prochaska, and the rest of their research team the first to localize an FRB that was not repeating. By the following year, the team had localized about five of them. By 2020, they had published a paper in Nature declaring that the FRBs had let them count up the universe’s missing baryons.
The centerpiece of the paper’s argument was something called the dispersion measure—a number that reflects how much an FRB’s light has been smeared by all the free electrons along our line of sight. In general, the farther an FRB travels, the higher the dispersion measure should be. Armed with both the travel distance (the redshift) and the dispersion measure for a number of FRBs, the researchers found they could extrapolate the total density of particles in the universe. J-P Macquart, the paper’s lead author, believed that the relationship between dispersion measure and FRB distance was predictable and could be applied to map the universe.
As a leader in the field and a key player in the advancement of FRB research, Macquart would have been interviewed for this piece. But he died of a heart attack one week after the paper was published, at the age of 45. FRB researchers began to call the relationship between dispersion and distance the “Macquart relation,” in honor of his memory and his push for the groundbreaking idea that FRBs could be used for cosmology.
Proving that the Macquart relation would hold at greater distances became not just a scientific quest but also an emotional one.
“I remember thinking that I know something about the universe that no one else knows.”
The researchers knew that the ASKAP telescope was capable of detecting bursts from very far away—they just needed to find one. Whenever the telescope detected an FRB, Ryder was tasked with helping to determine where it had originated. It took much longer than he would have liked. But one morning in July 2022, after many months of frustration, Ryder downloaded the newest data email from the European Southern Observatory and began to scroll through the spectrum data. Scrolling, scrolling, scrolling—and then there it was: light from 8 billion years ago, or a redshift of one, symbolized by two very close, bright lines on the computer screen, showing the optical emissions from oxygen. “I remember thinking that I know something about the universe that no one else knows,” he says. “I wanted to jump onto a Slack and tell everyone, but then I thought: No, just sit here and revel in this. It has taken a lot to get to this point.”
With the October 2023 Science paper, the team had basically doubled the distance baseline for the Macquart relation, honoring Macquart’s memory in the best way they knew how. The distance jump was significant because Ryder and the others on his team wanted to confirm that their work would hold true even for FRBs whose light comes from so far away that it reflects a much younger universe. They also wanted to establish that it was possible to find FRBs at this redshift, because astronomers need to collect evidence about many more like this one in order to create the cosmological map that motivates so much FRB research.
“It’s encouraging that the Macquart relation does still seem to hold, and that we can still see fast radio bursts coming from those distances,” Ryder said. “We assume that there are many more out there.”
Mapping the cosmic web
The missing stuff that lies between galaxies, which should contain the majority of the matter in the universe, is often called the cosmic web. The diffuse gases aren’t floating like random clouds; they’re strung together more like a spiderweb, a complex weaving of delicate filaments that stretches as the galaxies at their nodes grow and shift. This gas probably escaped from galaxies into the space beyond when the galaxies first formed, shoved outward by massive explosions.
“We don’t understand how gas is pushed in and out of galaxies. It’s fundamental for understanding how galaxies form and evolve,” says Kiyoshi Masui, the director of MIT’s Synoptic Radio Lab. “We only exist because stars exist, and yet this process of building up the building blocks of the universe is poorly understood … Our ability to model that is the gaping hole in our understanding of how the universe works.”
Astronomers are also working to build large-scale maps of galaxies in order to precisely measure the expansion of the universe. But the cosmological modeling underway with FRBs should create a picture of invisible gasses between galaxies, one that currently does not exist. To build a three-dimensional map of this cosmic web, astronomers will need precise data on thousands of FRBs from regions near Earth and from very far away, like the FRB at redshift one. “Ultimately, fast radio bursts will give you a very detailed picture of how gas gets pushed around,” Masui says. “To get to the cosmological data, samples have to get bigger, but not a lot bigger.”
That’s the task at hand for Masui, who leads a team searching for FRBs much closer to our galaxy than the ones found by the Australian-led collaboration. Masui’s team conducts FRB research with the CHIME telescope in British Columbia, a nontraditional radio telescope with a very wide field of view and focusing reflectors that look like half-pipes instead of dishes. CHIME (short for “Canadian Hydrogen Intensity Mapping Experiment)” has no moving parts and is less reliant on mirrors than a traditional telescope (focusing light in only one direction rather than two), instead using digital techniques to process its data. CHIME can use its digital technology to focus on many places at once, creating a 200-square-degree field of view compared with ASKAP’s 30-degree one. Masui likened it to a mirror that can be focused on thousands of different places simultaneously.
Because of this enormous field of view, CHIME has been able to gather data on thousands of bursts that are closer to the Milky Way. While CHIME cannot yet precisely locate where they are coming from the way that ASKAP can (the telescope is much more compact, providing lower resolution), Masui is leading the effort to change that by building three smaller versions of the same telescope in British Columbia; Green Bank, West Virginia; and Northern California. The additional data provided by these telescopes, the first of which will probably be collected sometime this year, can be combined with data from the original CHIME telescope to produce location information that is about 1,000 times more precise. That should be detailed enough for cosmological mapping.
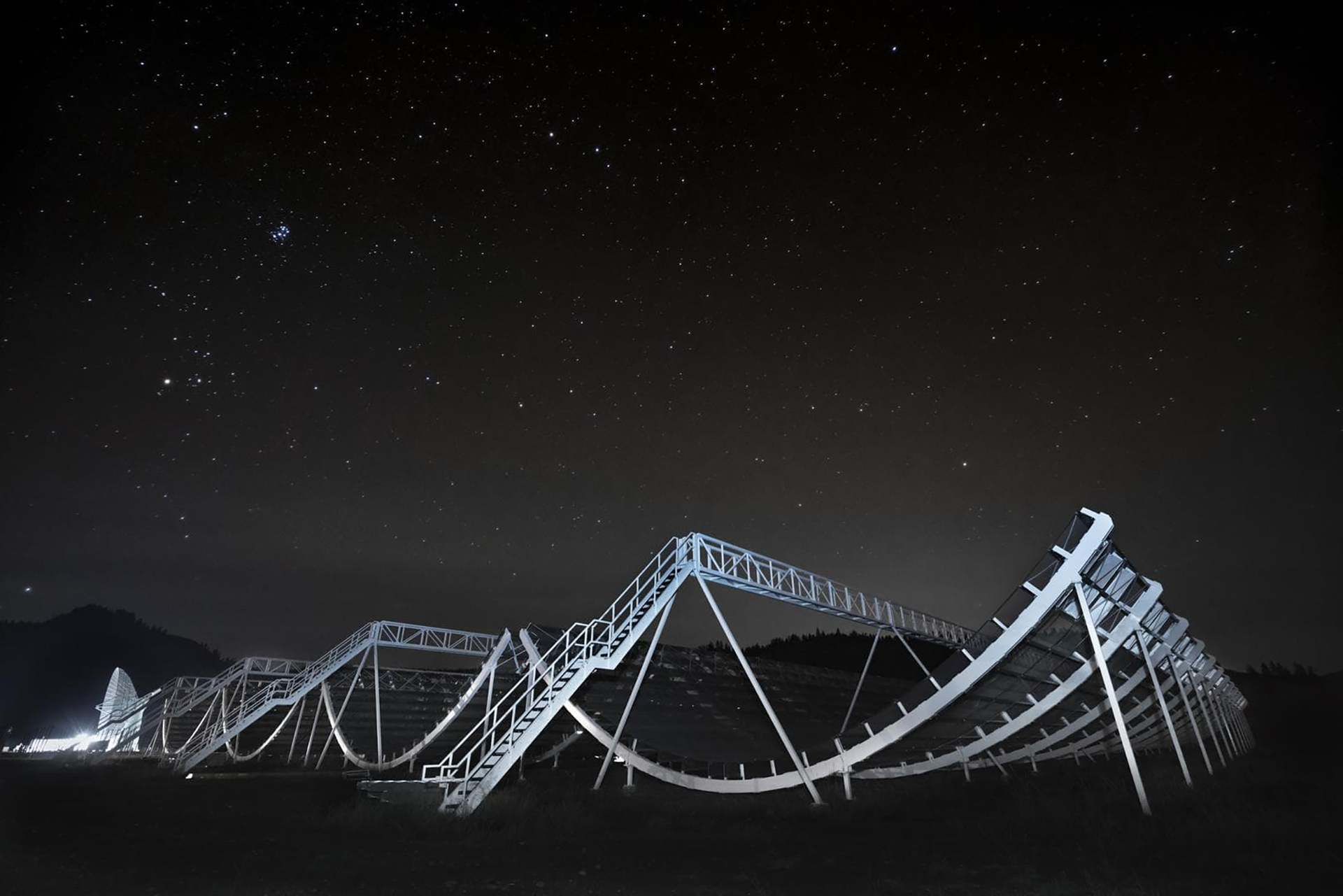
Telescope technology is improving so fast that the quest to gather enough FRB samples from different parts of the universe for a cosmological map could be finished within the next 10 years. In addition to CHIME, the BURSTT radio telescope in Taiwan should go online this year; the CHORD telescope in Canada, designed to surpass CHIME, should begin operations in 2025; and the Deep Synoptic Array in California could transform the field of radio astronomy when it’s finished, which is expected to happen sometime around the end of the decade.
And at ASKAP, Bannister is building a new tool that will quintuple the sensitivity of the telescope, beginning this year. If you can imagine stuffing a million people simultaneously watching uncompressed YouTube videos into a box the size of a fridge, that’s probably the easiest way to visualize the data handling capabilities of this new processor, called a field-programmable gate array, which Bannister is almost finished programming. He expects the new device to allow the team to detect one new FRB each day.
With all the telescopes in competition, Bannister says, “in five or 10 years’ time, there will be 1,000 new FRBs detected before you can write a paper about the one you just found … We’re in a race to make them boring.”
Prochaska is so confident FRBs will finally give us the cosmological map he’s been working toward his entire life that he’s started studying for a degree in oceanography. Once astronomers have measured distances for 1,000 of the bursts, he plans to give up the work entirely.
“In a decade, we could have a pretty decent cosmological map that’s very precise,” he says. “That’s what the 1,000 FRBs are for—and I should be fired if we don’t.”
Unlike most scientists, Prochaska can define the end goal. He knows that all those FRBs should allow astronomers to paint a map of the invisible gases in the universe, creating a picture of how galaxies evolve as gases move outward and then fall back in. FRBs will grant us an understanding of the shape of the universe that we don’t have today—even if the mystery of what makes them endures.
Anna Kramer is a science and climate journalist based in Washington, D.C.