Is this the end of animal testing?
In a clean room in his lab, Sean Moore peers through a microscope at a bit of intestine, its dark squiggles and rounded structures standing out against a light gray background. This sample is not part of an actual intestine; rather, it’s human intestinal cells on a tiny plastic rectangle, one of 24 so-called “organs on chips” his lab bought three years ago.
Moore, a pediatric gastroenterologist at the University of Virginia School of Medicine, hopes the chips will offer answers to a particularly thorny research problem. He studies rotavirus, a common infection that causes severe diarrhea, vomiting, dehydration, and even death in young children. In the US and other rich nations, up to 98% of the children who are vaccinated against rotavirus develop lifelong immunity. But in low-income countries, only about a third of vaccinated children become immune. Moore wants to know why.
His lab uses mice for some protocols, but animal studies are notoriously bad at identifying human treatments. Around 95% of the drugs developed through animal research fail in people. Researchers have documented this translation gap since at least 1962. “All these pharmaceutical companies know the animal models stink,” says Don Ingber, founder of the Wyss Institute for Biologically Inspired Engineering at Harvard and a leading advocate for organs on chips. “The FDA knows they stink.”
But until recently there was no other option. Research questions like Moore’s can’t ethically or practically be addressed with a randomized, double-blinded study in humans. Now these organs on chips, also known as microphysiological systems, may offer a truly viable alternative. They look remarkably prosaic: flexible polymer rectangles about the size of a thumb drive. In reality they’re triumphs of bioengineering, intricate constructions furrowed with tiny channels that are lined with living human tissues. These tissues expand and contract with the flow of fluid and air, mimicking key organ functions like breathing, blood flow, and peristalsis, the muscular contractions of the digestive system.
More than 60 companies now produce organs on chips commercially, focusing on five major organs: liver, kidney, lung, intestines, and brain. They’re already being used to understand diseases, discover and test new drugs, and explore personalized approaches to treatment.
As they continue to be refined, they could solve one of the biggest problems in medicine today. “You need to do three things when you’re making a drug,” says Lorna Ewart, a pharmacologist and chief scientific officer of Emulate, a biotech company based in Boston. “You need to show it’s safe. You need to show it works. You need to be able to make it.”
All new compounds have to pass through a preclinical phase, where they’re tested for safety and effectiveness before moving to clinical trials in humans. Until recently, those tests had to run in at least two animal species—usually rats and dogs—before the drugs were tried on people.
But in December 2022, President Biden signed the FDA Modernization Act, which amended the original FDA Act of 1938. With a few small word changes, the act opened the door for non-animal-based testing in preclinical trials. Anything that makes it faster and easier for pharmaceutical companies to identify safe and effective drugs means better, potentially cheaper treatments for all of us.
Moore, for one, is banking on it, hoping the chips help him and his colleagues shed light on the rotavirus vaccine responses that confound them. “If you could figure out the answer,” he says, “you could save a lot of kids’ lives.”
While many teams have worked on organ chips over the last 30 years, the OG in the field is generally acknowledged to be Michael Shuler, a professor emeritus of chemical engineering at Cornell. In the 1980s, Shuler was a math and engineering guy who imagined an “animal on a chip,” a cell culture base seeded with a variety of human cells that could be used for testing drugs. He wanted to position a handful of different organ cells on the same chip, linked to one another, which could mimic the chemical communication between organs and the way drugs move through the body. “This was science fiction,” says Gordana Vunjak-Novakovic, a professor of biomedical engineering at Columbia University whose lab works with cardiac tissue on chips. “There was no body on a chip. There is still no body on a chip. God knows if there will ever be a body on a chip.”
Shuler had hoped to develop a computer model of a multi-organ system, but there were too many unknowns. The living cell culture system he dreamed up was his bid to fill in the blanks. For a while he played with the concept, but the materials simply weren’t good enough to build what he imagined.
“You can force mice to menstruate, but it’s not really menstruation. You need the human being.”
Linda Griffith, founding professor of biological engineering at MIT and a 2006 recipient of a MacArthur “genius grant”
He wasn’t the only one working on the problem. Linda Griffith, a founding professor of biological engineering at MIT and a 2006 recipient of a MacArthur “genius grant,” designed a crude early version of a liver chip in the late 1990s: a flat silicon chip, just a few hundred micrometers tall, with endothelial cells, oxygen and liquid flowing in and out via pumps, silicone tubing, and a polymer membrane with microscopic holes. She put liver cells from rats on the chip, and those cells organized themselves into three-dimensional tissue. It wasn’t a liver, but it modeled a few of the things a functioning human liver could do. It was a start.
Griffith, who rides a motorcycle for fun and speaks with a soft Southern accent, suffers from endometriosis, an inflammatory condition where cells from the lining of the uterus grow throughout the abdomen. She’s endured decades of nausea, pain, blood loss, and repeated surgeries. She never took medical leaves, instead loading up on Percocet, Advil, and margaritas, keeping a heating pad and couch in her office—a strategy of necessity, as she saw no other choice for a working scientist. Especially a woman.
And as a scientist, Griffith understood that the chronic diseases affecting women tend to be under-researched, underfunded, and poorly treated. She realized that decades of work with animals hadn’t done a damn thing to make life better for women like her. “We’ve got all this data, but most of that data does not lead to treatments for human diseases,” she says. “You can force mice to menstruate, but it’s not really menstruation. You need the human being.”
Or, at least, the human cells. Shuler and Griffith, and other scientists in Europe, worked on some of those early chips, but things really kicked off around 2009, when Don Ingber’s lab in Cambridge, Massachusetts, created the first fully functioning organ on a chip. That “lung on a chip” was made from flexible silicone rubber, lined with human lung cells and capillary blood vessel cells that “breathed” like the alveoli—tiny air sacs—in a human lung. A few years later Ingber, an MD-PhD with the tidy good looks of a younger Michael Douglas, founded Emulate, one of the earliest biotech companies making microphysiological systems. Since then he’s become a kind of unofficial ambassador for in vitro technologies in general and organs on chips in particular, giving hundreds of talks, scoring millions in grant money, repping the field with scientists and laypeople. Stephen Colbert once ragged on him after the New York Times quoted him as describing a chip that “walks, talks, and quacks like a human vagina,” a quote Ingber says was taken out of context.
Ingber began his career working on cancer. But he struggled with the required animal research. “I really didn’t want to work with them anymore, because I love animals,” he says. “It was a conscious decision to focus on in vitro models.” He’s not alone; a growing number of young scientists are speaking up about the distress they feel when research protocols cause pain, trauma, injury, and death to lab animals. “I’m a master’s degree student in neuroscience and I think about this constantly. I’ve done such unspeakable, horrible things to mice all in the name of scientific progress, and I feel guilty about this every day,” wrote one anonymous student on Reddit. (Full disclosure: I switched out of a psychology major in college because I didn’t want to cause harm to animals.)
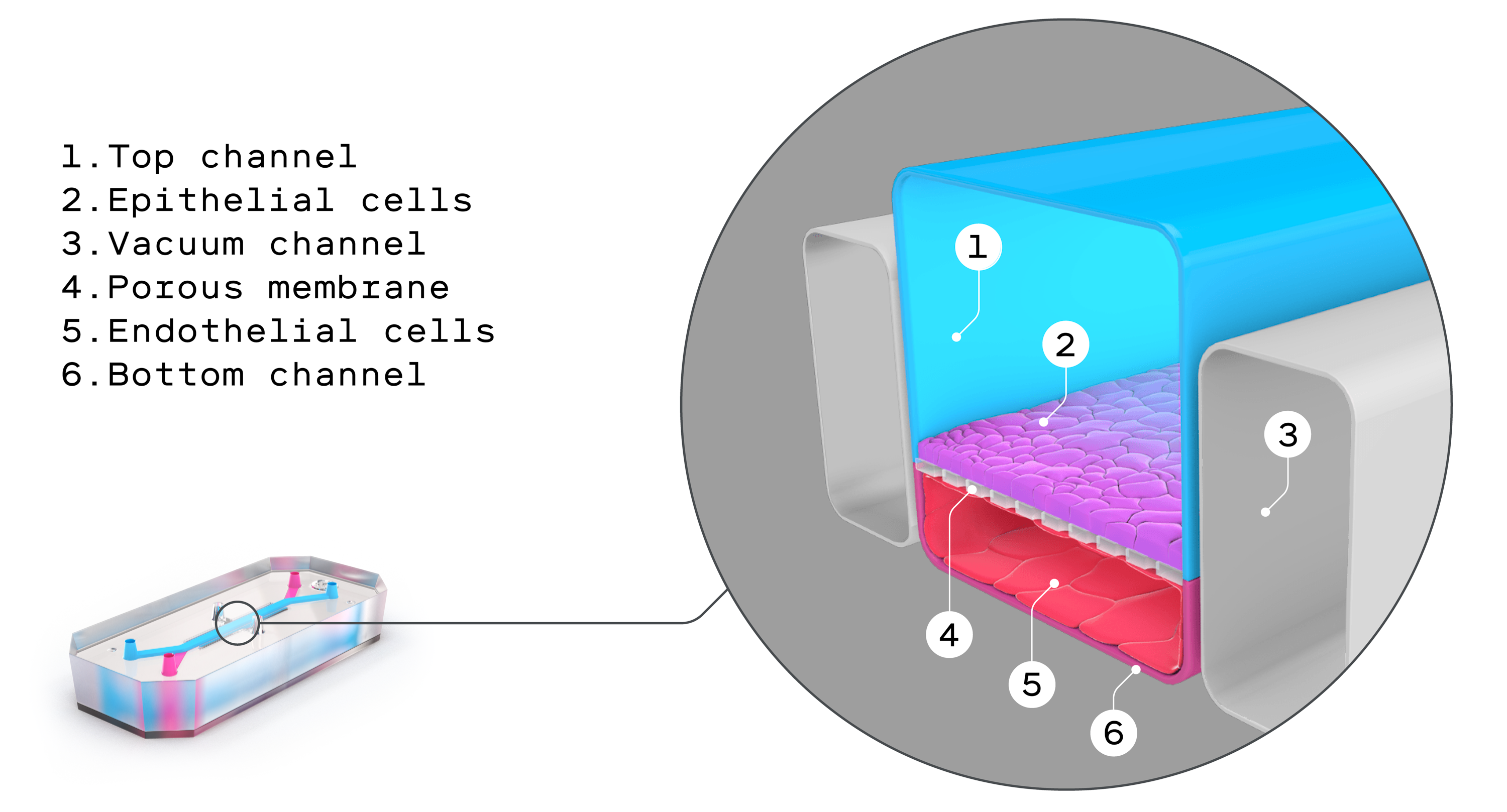
Taking an undergraduate art class led Ingber to an epiphany: mechanical forces are just as important as chemicals and genes in determining the way living creatures work. On a shelf in his office he still displays a model he built in that art class, a simple construction of sticks and fishing line, which helped him realize that cells pull and twist against each other. That realization foreshadowed his current work and helped him design dynamic microfluidic devices that incorporated shear and flow.
Ingber coauthored a 2022 paper that’s sometimes cited as a watershed in the world of organs on chips. Researchers used Emulate’s liver chips to reevaluate 27 drugs that had previously made it through animal testing and had then gone on to kill 242 people and necessitate more than 60 liver transplants. The liver chips correctly flagged problems with 22 of the 27 drugs, an 87% success rate compared with a 0% success rate for animal testing. It was the first time organs on chips had been directly pitted against animal models, and the results got a lot of attention from the pharmaceutical industry. Dan Tagle, director of the Office of Special Initiatives for the National Center for Advancing Translational Sciences (NCATS), estimates that drug failures cost around $2.6 billion globally each year. The earlier in the process failing compounds can be weeded out, the more room there is for other drugs to succeed.
“The capacity we have to test drugs is more or less fixed in this country,” says Shuler, whose company, Hesperos, also manufactures organs on chips. “There are only so many clinical trials you can do. So if you put a loser into the system, that means something that could have won didn’t get into the system. We want to change the success rate from clinical trials to a much higher number.”
In 2011, the National Institutes of Health established NCATS and started investing in organs on chips and other in vitro technologies. Other government funders, like the Defense Advanced Research Projects Agency and the Food and Drug Administration, have followed suit. For instance, NIH recently funded NASA scientists to send heart tissue on chips into space. Six months in low gravity ages the cardiovascular system 10 years, so this experiment lets researchers study some of the effects of aging without harming animals or humans.
Scientists have made liver chips, brain chips, heart chips, kidney chips, intestine chips, and even a female reproductive system on a chip (with cells from ovaries, fallopian tubes, and uteruses that release hormones and mimic an actual 28-day menstrual cycle). Each of these chips exhibits some of the specific functions of the organs in question. Cardiac chips, for instance, contain heart cells that beat just like heart muscle, making it possible for researchers to model disorders like cardiomyopathy.
Shuler thinks organs on chips will revolutionize the world of research for rare diseases. “It is a very good model when you don’t have enough patients for normal clinical trials and you don’t have a good animal model,” he says. “So it’s a way to get drugs to people that couldn’t be developed in our current pharmaceutical model.” Shuler’s own biotech company used organs on chips to test a potential drug for myasthenia gravis, a rare neurological disorder. In 2022,the FDA approved the drug for clinical trials based on that data—one of six Hesperos drugs that have so far made it to that stage.
Each chip starts with a physiologically based pharmacokinetic model, known as a PBPK model—a mathematical expression of how a chemical compound behaves in a human body. “We try and build a physical replica of the mathematical model of what really occurs in the body,” explains Shuler. That model guides the way the chip is designed, re-creating the amount of time a fluid or chemical stays in that particular organ—what’s known as the residence time. “As long as you have the same residence time, you should get the same response in terms of chemical conversion,” he says.
Tiny channels on each chip, each between 10 and 100 microns in diameter, help bring fluids and oxygen to the cells. “When you get down to less than one micron, you can’t use normal fluid dynamics,” says Shuler. And fluid dynamics matters, because if the fluid moves through the device too quickly, the cells might die; too slowly, and the cells won’t react normally.
Chip technology, while sophisticated, has some downsides. One of them is user friendliness. “We need to get rid of all this tubing and pumps and make something that’s as simple as a well plate for culturing cells,” says Vunjak-Novakovic. Her lab and others are working on simplifying the design and function of such chips so they’re easier to operate and are compatible with robots, which do repetitive tasks like pipetting in many labs.
Cost and sourcing can also be challenging. Emulate’s base model, which looks like a simple rectangular box from the outside,starts at around $100,000 and rises steeply from there. Most human cells come from commercial suppliers that arrange for donations from hospital patients. During the pandemic, when people had fewer elective surgeries, many of those sources dried up. As microphysiological systems become more mainstream, finding reliable sources of human cells will be critical.
“As your confidence in using the chips grows, you might say, Okay, we don’t need two animals anymore— we could go with chip plus one animal.”
Lorna Ewart, Chief Scientific Officer, Emulate
Another challenge is that every company producing organs on chips uses its own proprietary methods and technologies. Ingber compares the landscape to the early days of personal computing, when every company developed its own hardware and software, and none of them meshed well. For instance, the microfluidic systems in Emulate’s intestine chips are fueled by micropumps, while those made by Mimetas, another biotech company, use an electronic rocker and gravity to circulate fluids and air. “This is not an academic lab type of challenge,” emphasizes Ingber. “It’s a commercial challenge. There’s no way you can get the same results anywhere in the world with individual academics making [organs on chips], so you have to have commercialization.”
Namandje Bumpus, the FDA’s chief scientist, agrees. “You can find differences [in outcomes] depending even on what types of reagents you’re using,” she says. Those differences mean research can’t be easily reproduced, which diminishes its validity and usefulness. “It would be great to have some standardization,” she adds.
On the plus side, the chip technology could help researchers address some of the most deeply entrenched health inequities in science. Clinical trials have historically recruited white men, underrepresenting people of color, women (especially pregnant and lactating women), the elderly, and other groups. And treatments derived from those trials all too often fail in members of those underrepresented groups, as in Moore’s rotavirus vaccine mystery. “With organs on a chip, you may be able to create systems by which you are very, very thoughtful—where you spread the net wider than has ever been done before,” says Moore.
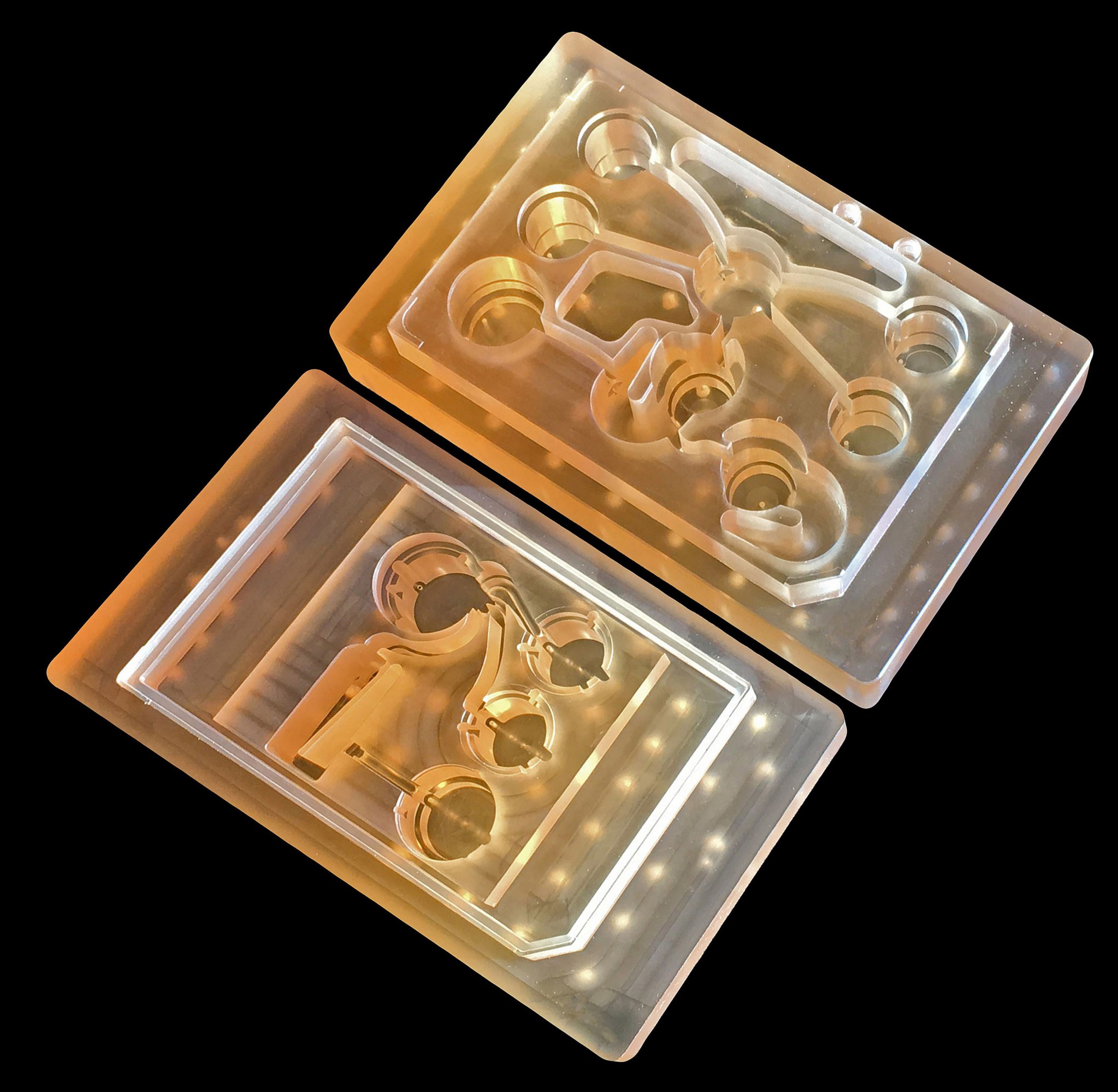
Another advantage is that chips will eventually reduce the need for animals in the lab even as they lead to better human outcomes. “There are aspects of animal research that make all of us uncomfortable, even people that do it,” acknowledges Moore. “The same values that make us uncomfortable about animal research are also the same values that make us uncomfortable with seeing human beings suffer with diseases that we don’t have cures for yet. So we always sort of balance that desire to reduce suffering in all the forms that we see it.”
Lorna Ewart, who spent 20 years at the pharma giant AstraZeneca before joining Emulate, thinks we’re entering a kind of transition time in research, in which scientists use in vitro technologies like organs on chips alongside traditional cell culture methods and animals. “As your confidence in using the chips grows, you might say, Okay, we don’t need two animals anymore—we could go with chip plus one animal,” she says.
In the meantime, Sean Moore is excited about incorporating intestine chips more and more deeply into his research. His lab has been funded by the Gates Foundation to do what he laughingly describes as a bake-off between intestine chips made by Emulate and Mimetas. They’re infecting the chips with different strains of rotavirus to try to identify the pros and cons of each company’s design. It’s too early for any substantive results, but Moore says he does have data showing that organ chips are a viable model for studying rotavirus infection. That could ultimately be a real game-changer in his lab and in labs around the world.
“There’s more players in the space right now,” says Moore. “And that competition is going to be a healthy thing.”
Harriet Brown writes about health, medicine, and science. Her most recent book is Shadow Daughter: A Memoir of Estrangement. She’s a professor of magazine, news, and digital journalism at Syracuse University’s Newhouse School.